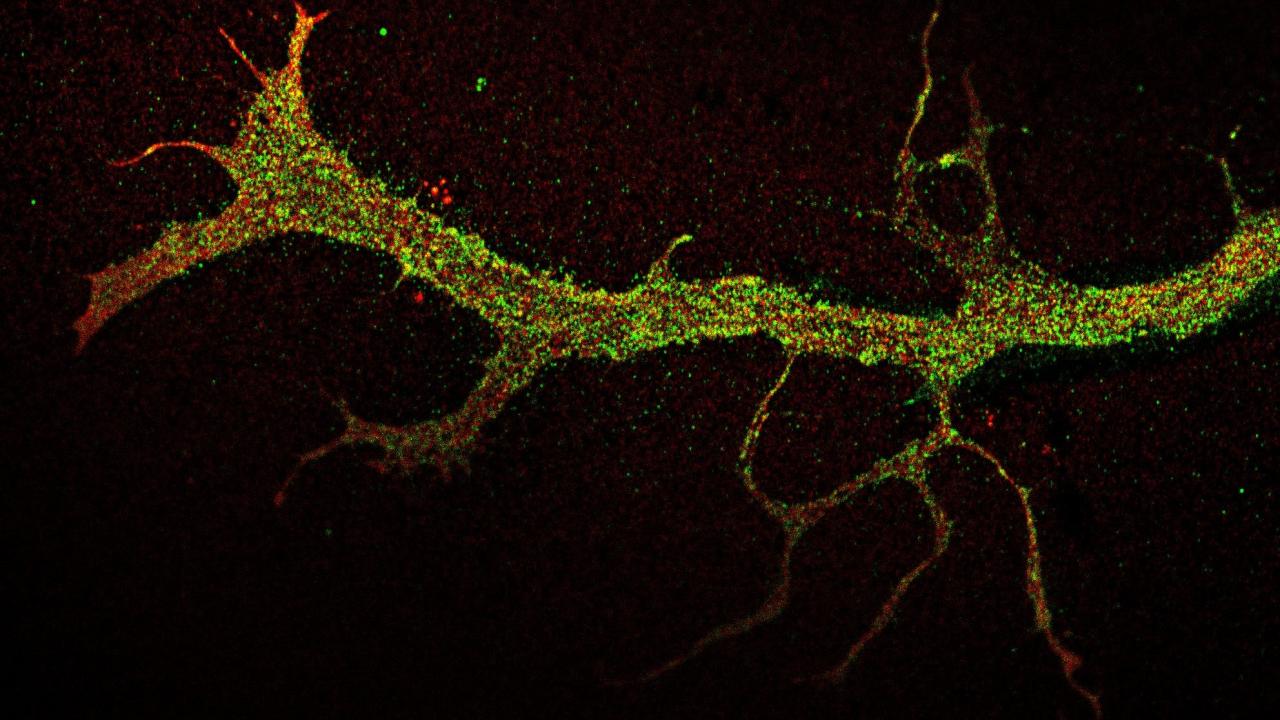
Breaking Down the Gut-Brain Divide
Our researchers are taking the phrase “follow your gut” to a whole new level
Inside a minuscule chamber filled with liquid, barely visible to the human eye, a neuron probes the unknown. Thin, tail-like projections stretch from its cell body into the empty space, collecting and communicating information about its environment in electrical and chemical impulses. It finds itself hemmed in on every side.
Suddenly, the projections, known as neurites, discover something: a small passageway large enough to fit through. The neurites press on, taking residence in another chamber just out of reach from the soma, or the neuron’s cell body.
In this wandering, compartmentalized form, there’s immense promise. It is a window into the anatomical layout of neurons inside of a device, allowing researchers to expose their neurites and soma to different electrical, biochemical and even microorganismal signals and learn how these cues may influence neuronal activity and, ultimately, the whole body.
This type of technology, referred to as microfluidic devices or organs-on-chips, has emerged as a method for unparalleled insight into how human organs work, yet no one has been able to use it to model the sensory nerves that connect the gut and the brain. Until now.
Erkin Şeker, a professor of electrical and computer engineering at the University of California, Davis, and his lab have developed a microfluidic device that allows researchers to model the neuronal communication between the gastrointestinal tract and the central nervous system, or CNS. It’s an essential advancement in an interdisciplinary, collaborative research initiative helmed by Şeker to understand the gut-brain axis — and eventually to improve outcomes for conditions related to the gut, like Crohn’s disease and mental health issues.
Gut Check
The gut-brain axis, or GBA, is the two-way communication system between the brain and the body’s digestive system. It spans the 100 billion neurons that run along and through the brain and spinal cord to form the central nervous system (which, by itself, matches the number of stars in the Milky Way galaxy) and the roughly 500 million neurons that make up a mesh-like structure called the enteric nervous system in the gut. It also features the 100 trillion microbiota that call the gut home.
This complex relationship allows the brain to influence processes like digestion and the gut to send signals to the brain, like the state of being full and phrases like “butterflies in my stomach.” Yet, this connection is responsible for more than satiety and fluttery feelings.
Research from the past few decades continues to show that the gastrointestinal system holds a tremendous amount of sway over the brain, particularly because of the microbiota lining the gut. These microorganisms have connections to dementia and the neurologic development of newborn babies.
The reasons why, however, are not well understood, due to the myriad molecules and physiological processes that constantly run throughout the nerves in our gut and brain. This is why it is so critical to have a method for modeling the GBA: researchers can finally isolate specific components and processes of the axis to determine what impacts they have on human health.
Compartmentalizing Knowledge
Modeling the entirety of the GBA in one device isn’t necessary. Doing that would essentially replicate the complexity within the human body, making it harder to isolate which physiological processes are responsible for positive or negative health outcomes.
“The brain, the gut and where the cell bodies are all have different microenvironments to them,” said Şeker. “If you were to put everything in one chamber, you wouldn’t be able to specify what types of chemicals each part is exposed to.”
In other words, it’s better to piece out this puzzle one by one.
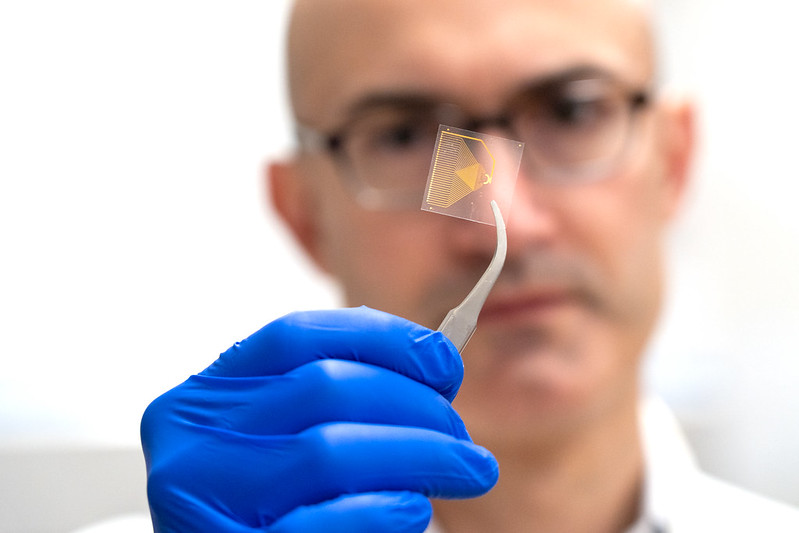
In his modeling device, Şeker has isolated the mechanisms and microenvironments peculiar to the sensory arm of the vagus nerve, or the nerve fibers that relay information from the peripheral organs like the heart, lungs and gut to the brain. Specifically, his device recapitulates — or forces an anatomical recreation — of the nodose ganglion.
The nodose ganglion is the part of the vagus nerve where the cell bodies develop and is located somewhere behind the jaw and just below the skull. These cell bodies then develop neurites that extend downward toward the gastrointestinal tract, as well as toward the brain, forming a communication network with the gut and brain. To accurately model this relationship, Şeker has designed his microfluidic device into two chambers.
The first chamber simulates the sensory endings of the vagus nerve. In the center is a smaller, circular chamber where a culture of vagal afferent neuron cell bodies — or VANs, neurons that relay the signals picked up at the nerve endings toward the CNS — are placed. This mimics the nodose ganglion where all the cell bodies are found. A small corridor, fit for neurites, leads out from this space into an expansive chamber that imitates the region just beneath the gut lining, where enteric neurons and VAN terminals reside.
This segmentation allows researchers to independently expose the neurites to different molecules to see how they react. The use of VANs is also critical for this device, as these neurons only carry information to the brain and never back to the gut.
“VANs not only respond to chemical signals generated by the body, such as satiety-inducing hormones, but also to molecules produced by gut microbes,” Şeker said. “This is why the ability to intentionally expose the VAN endings to specific molecules is so important to be able to tease out their individual and collective influences on VAN activity.”
In addition, with this microfluidic platform, Şeker and his team have demonstrated VANs’ ability to transport a fluorescently labeled protein from the neurites in the gut to the soma and additional neurites emanating from cell bodies. This makes it far easier to observe how things — for example, pathogenic proteins that may result in neurodegenerative diseases — are transmitted from gut microbiota to the brain via the vagus nerve, an advancement that can also be thought of as enabling researchers to follow the gut unlike ever before.
Two specific examples of how this microfluidic platform could be used are as a screening device to see how medications alter gut health and to study diseases where gut inflammation is important, such as Chron’s disease.
“What generally happens when the gut is inflamed is that the intestinal barrier becomes more permeable,” he said. “The bacterial products that should stay in the intestine begin to leak through the intestinal barrier and influence neuronal function. So, with this microfluidic platform, you can envision setting up a model where you add those bacterial products and then try out different therapeutics to see if it can normalize the neuronal activity.”
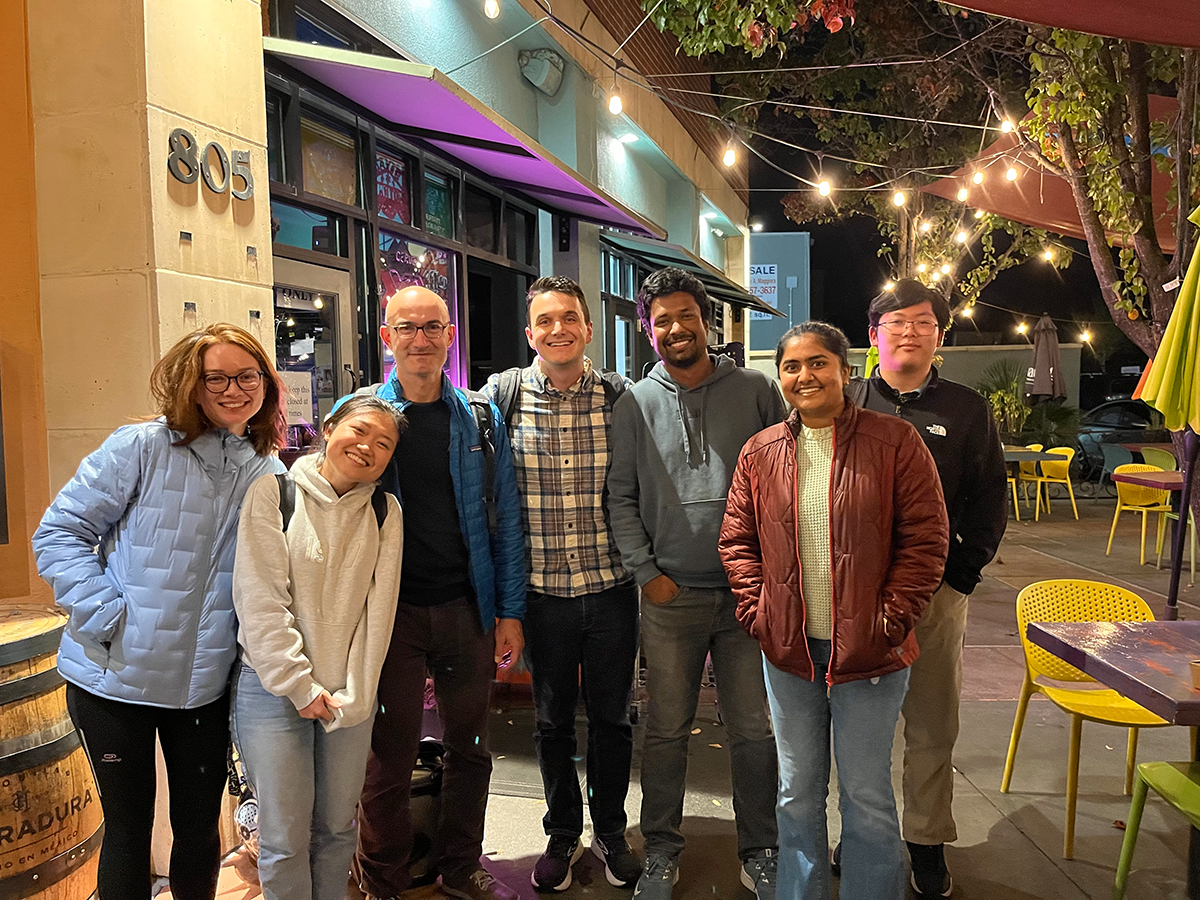
The thing is, while this platform stands on its own to greatly increase science’s ability to answer complicated questions about the GBA and its potential influence on health and disease, it is missing the vagus nerve’s connection to the brain. This is where Şeker’s other microfluidic device comes into play.
This second platform features a unique neuronal tri-culture Şeker and his team developed with Pamela Lein, a professor of neurotoxicology and chair of the Department of Molecular Biosciences in the School of Veterinary Medicine.
The tri-culture features microglia, astrocytes and neurons, three cell types that play an important role in the CNS. This model is particularly useful for studying how microglia — a sensitive, historically tough-to-research cell that is the CNS’s first line of defense against foreign invaders and housekeeping to maintain a healthy brain environment — is responsible for orchestrating inflammation in the brain, which is present in many neurological disorders.
While still in development, Şeker is working to connect this device to his model of the vagus nerve. The thought is the chamber that replicates the nodose ganglion will be in the middle and feature two small corridors on either side, with one extending into the chamber that mirrors the gut and the other the brain.
In this interconnected structure, the modeling technologies will more closely resemble the anatomical differentiation of the CNS and vagal afferent neurons that bridge the enteric system to the CNS. Moreover, their potential to explain, once and for all, how the GBA impacts human health will be supercharged.
A Collaborative Culture
The advancements Şeker and his team have made to model the GBA are thanks to collaborations both inside and outside the College of Engineering. In fact, the whole idea behind the initiative can be traced to a cross-disciplinary partnership.
In 2012, Şeker and a team of collaborators, from food scientists to plant pathologists and chemical engineers, received funding from UC Davis as part of a project that looked at ways to detect nucleic acid for improved food safety. Şeker’s contribution to the team was developing electrochemical sensors and microfluidic devices, not too dissimilar from the GBA modeling technology.
Fast forward to 2018, a Microbiome Special Research Program was established at UC Davis, and there was a call for pilot proposals. This, coupled with the nucleic acid research project he was working on, led him to his light bulb moment.
“We were working on these microfluidic platforms for nucleic acids, and I said to the team, ‘Hey, maybe we could actually use this to model the gut-brain axis,’” Şeker said. “Automatically, we felt that it would be really interesting because no one had ever done it before.”
He worked with Maria Marco, a co-investigator on the nucleic acid detection project and professor of food science and technology who studies microbes in the gut and food ecosystems, to bring this idea to life. Marco also connected with Professor Emerita Helen Raybould of UC Davis School of Veterinary Medicine, who specializes in the function of neurons in the gastrointestinal tract and cells that form the intestinal barrier, to work on the project.
From these interconnections, they wrote the initial proposal to model the GBA that now steers Şeker’s research and has attracted extramural research funding from the National Institutes of Health.
Within the college, he frequently collaborates with professors from the Departments of Biomedical Engineering and Chemical Engineering for their expertise and insights into the GBA. The microfluidic technology that underpins the modeling technology would also be impossible without the college’s Center for Nano-MicroManufacturing, a cleanroom where Şeker and his team fabricate each device.
The most cross-collaborations happen, however, at the Center for Neuroengineering and Medicine, where Şeker is the co-director and the inaugural chair for the Designated Emphasis in Neuroengineering. Here, he collaborates with a diverse group of faculty members and graduate students whose interests range from psychology to neuroethics.
“The center has been an incubator for cross-disciplinary research and training and has attracted top-notch faculty and trainees to UC Davis,” Şeker said.
“Through these projects on the GBA, there are so many things that are new to me as an electrical engineer. I really value multidisciplinary research, because I think you begin to look at problems from different points of view and continue to expand your scientific knowledge by taking yourself outside of your comfort zone.”
Future Tracts
With the advancement of microfluidic devices that can model the vagus nerve and the brain, there are outstanding research questions that can finally be probed.
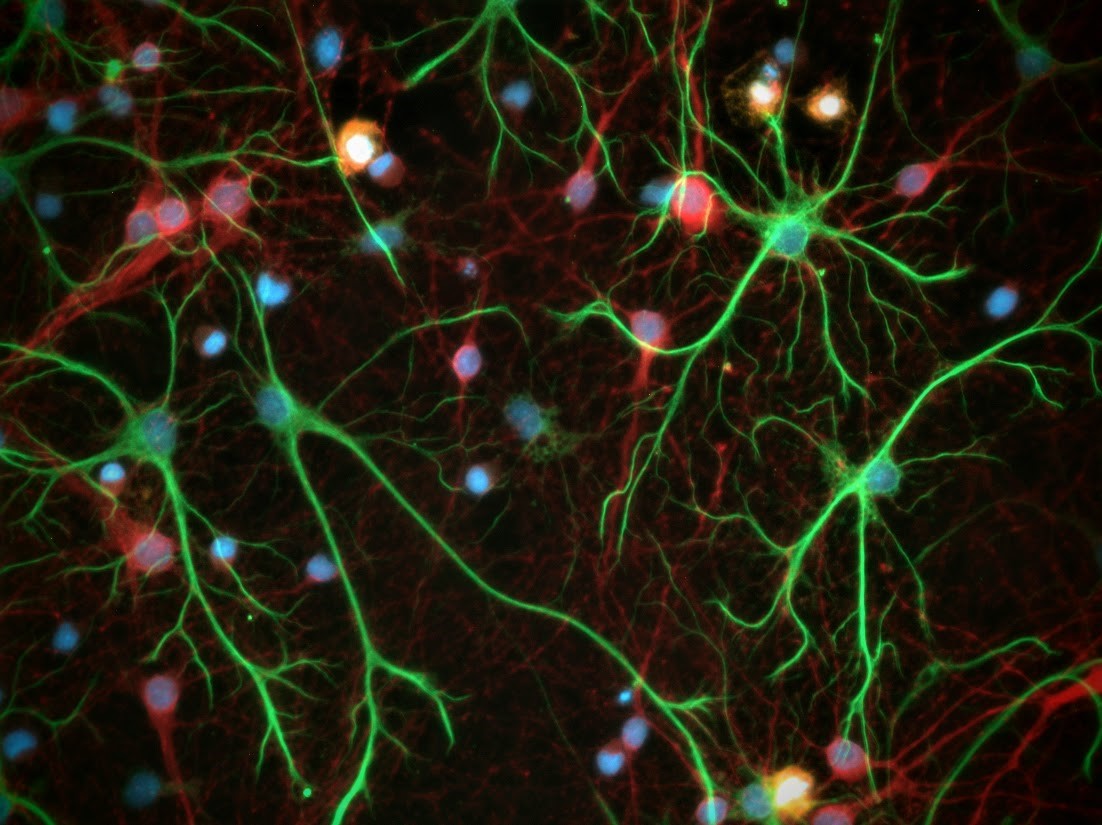
Şeker’s lab is exploring several research areas related to neurodegeneration and the neuroprotective role of microbiota-derived compounds, one of which has been supported with a College of Engineering Next Level Research Award.
“The versatility of the platform opened up many different collaboration opportunities across the campus for our laboratory, including researchers in the Department of Food Science and Technology and at the Alzheimer’s Disease Research Center,” Şeker said.
The technology will also help fuel new education and research opportunities for students in the College of Engineering.
Thanks to a nearly $1 million gift from Susan Ellis ’78 and her husband, Mark Linton, to the College of Engineering, the Center for Neuroengineering and Medicine will now be able to offer fellowships to students who are interested in engineering technologies — like Şeker’s microfluidic chambers — to advance knowledge on the inner workings of the GBA. This gift works in concert with the center’s NeuralStorm, an education and training program for undergraduate and graduate students to solve cross-disciplinary challenges in neuroengineering, led by Professor of Biomedical Engineering Karen Moxon and made possible through a grant from the National Science Foundation.
“These generous contributions to the center create an unparalleled educational environment to train the next generation of researchers on the exciting area of the gut-brain axis.”
Learn about how we advance human health